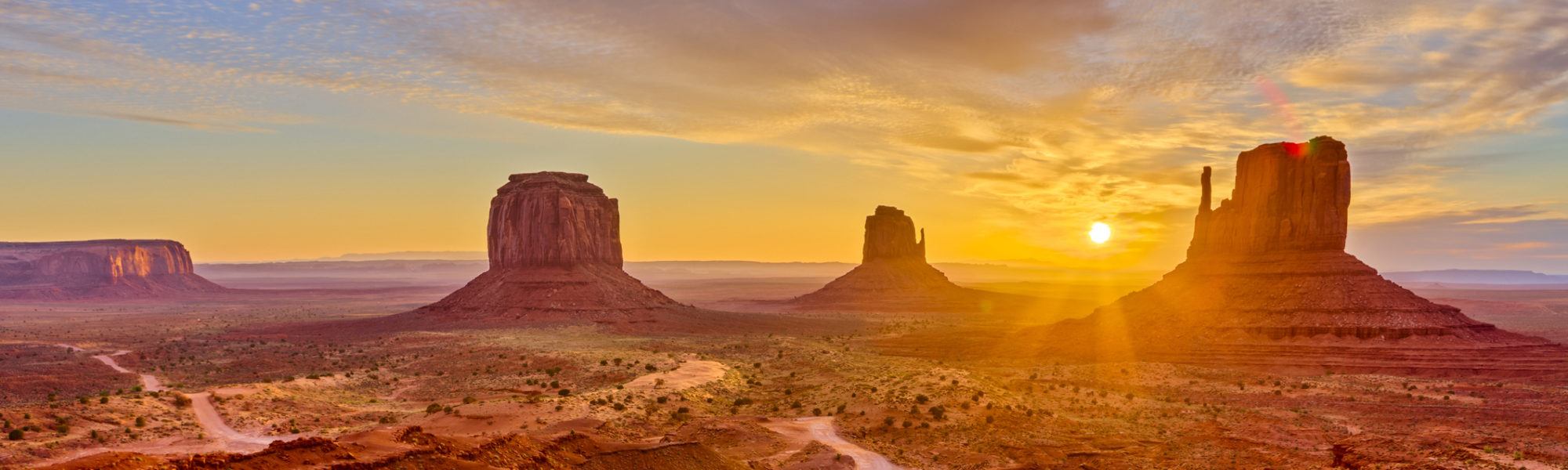
The Neural Control of Limb Movement*
Alan R. Gibson, PhD
*Courtesy of Alan R. Gibson, PhD
Division of Neurobiology, Barrow Neurological Institute, St. Joseph’s Hospital and Medical Center, Phoenix, Arizona
The Gibson Laboratory
Alan Gibson received his PhD in psychology from New York University working with Michael Gazzaniga who studied split brain humans. Gibson then studied the anatomy and physiology of cerebellar systems with Mitchell Glickstein at Brown University. Kris Horn obtained his PhD from the University of Utah where he studied the visual-vestibular system in the laboratory of S.W. Miller. Milton Pong completed his PhD at the University of Washington where he studied the pupillary light reflex in the laboratory of Albert Fuchs. Alton Marcello obtained his MD at Wright State University School of Medicine and joined our laboratory for one year of research training before entering a neurology residency at the University of Texas San Antonio. James Castano received his BS in biology from the Wooster Polytechnic Institute. He previously worked at the New England Primate Center and University of Rochester Department of Radiation Oncology.
The research in our laboratory is designed to understand how the nervous system controls limb movements. The cerebellum is a major neural structure involved in movement control, and our initial studies focused on understanding what limb movements were controlled by discharge of cerebellar output cells. We trained monkeys to make reaching movements with and without a grasping movement at the end of the reach. Cells in the cerebellar interpositus nuclei showed large increases in discharge rate when the monkeys reached and grasped an object, but they did not show increases to the reach alone. We believe that the interpositus nucleus plays a major role in shaping the hand prior to grasping an object. We then attempted to define regions of the brain important for directing the limb during reaching. In doing this, we identified several brainstem nuclei that receive input from the cerebellum and the basal ganglia. These regions are good candidates for control of reach direction and may mediate many of the motor disorders seen with basal gangliar disease, such as Parkinson’s disease. The progression of our research program demonstrates how understanding basic neural mechanisms for movement control can lead to new approaches in the clinical treatment of disease.
Key Words: basal ganglia, cerebellum, motor control, Parkinson’s disease, reach, red nucleus, spinal cord
Abbreviations used: GABA, gamma amino butyric acid; 6-OHDA, 6-hydroxydopamine; RNm, magnocellular red nucleus; RNp, parvicellular red nucleus; WGA-HRP, wheat germ agglutinin-horseradish peroxidase
Normally, reaching to grasp an object is performed quickly and easily without conscious effort. Yet, it is a complicated task of sensory-motor coordination, and even the most sophisticated machines cannot match the skill of an average human. Our laboratory has been studying the anatomy and physiology of brain regions important for controlling the limb during the reach to grasp.This article describes some of our findings that have helped advance the understanding of limb movement control. It also demonstrates how those findings have led to a research program that may help explain how diseases of the basal ganglia, such as Parkinson’s disease, affect movement control.
What is a Movement?
I was initially attracted to the study of movement control because the result, movement,could be seen and measured. As it turns out,this view was somewhat naïve. A movement can be described at many different levels, and there is no a priori way of knowing how a given brain region describes a movement. For example,a movement command could be as general as “pick up that object” or as specific as “activate a single muscle.” Abstract movement commands must be transformed to specific movement commands within the brain.We try to understand the control of movement by comparing the discharge patterns of individual cells in the brain with the movement being made. To do so successfully, we must first define what a movement is for the cell being studied.
Before moving to Barrow,I worked as a Research Associate in the laboratory of Dr. James Houk at Northwestern University Medical School. We were interested in determining neural codes for movement. Monkeys were trained to push and pull a handle against a variety of loads (e.g., inertial, constant force). After the monkeys were trained, we recorded from cells in a brainstem nucleus, the magnocellular red nucleus (RNm), while monkeys performed the push-pull task. We chose to record from RNm because this nucleus receives input from the cerebellum, a motor structure,and projects to neurons in the spinal cord. Presumably, the discharge patterns of RNm signals would be similar to those of spinal motor neurons,which directly activate muscles of the limb. Disappointingly, however, RNm neurons showed little change in discharge during the push-pull task regardless of the loading condition.[2] Yet, discharges from the same neurons increased considerably when the monkeys reached to grasp a raisin. The task had been inappropriate to study RNm neurons, but what was the appropriate task? The cells might be involved in control of the entire coordinated reaching movement. Alternatively, they might be involved in controlling some other aspect of reach to grasp not tapped by the push-pull device.
Discharge of Cerebellar Interpositus Neurons During Reaching and Grasping
At Barrow, Peter van Kan, (now a Professor at the University of Wisconsin), Kris Horn, and I decided to record from cerebellar interpositus cells while monkeys made two different types of limb movement. One movement would be similar to a reach to grasp but would not include a grasp at the completion of the movement. The other movement would be an entire reach to grasp. This comparison would reveal whether the reaching movement was an important factor for eliciting discharge or if some aspect associated with grasping was an important factor.We chose to study the cerebellar interpositus nucleus because these cells provide the major excitatory input to the RNm (Fig. 1).
The monkeys were trained to move a light articulated and counterbalanced arm between two target positions: one at the waist and the other at a position directly in front of the monkey. The limb moved in a trajectory similar to that of reaching from the waist to a point in front of the monkey at shoulder height. A small remotely operated drawer was mounted at each target position and loaded with a raisin.When the drawer was opened,the monkey would release the handle of the arm and reach and grasp the raisin. If coordination across the limb were important, both types of movement would elicit discharge from the interpositus cells. If grasping were important,only the reaches for the raisin would elicit discharge.
Neither upward nor downward movements of the handle produced a significant change in the rate of cell discharge; however, reaching to grasp a raisin considerably increased the rate of discharge on every trial (Fig.2).The increase in the rate of discharge before grasping was similar when the drawer was opened with the arm in the extended position with the hand next to the drawer, and discharge rate did not vary with direction of the reach.
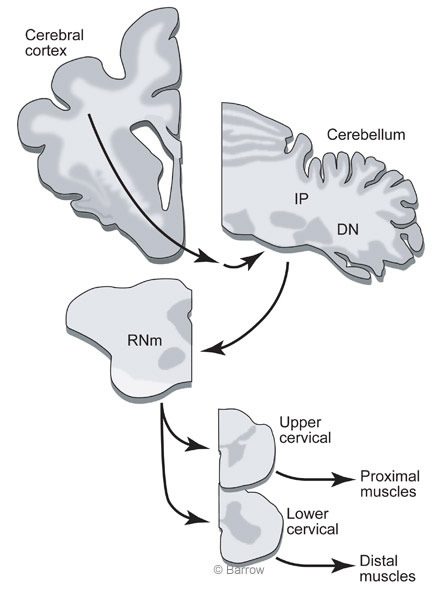
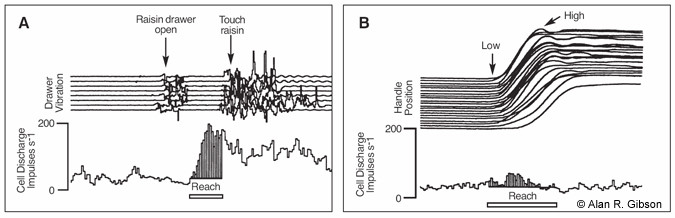
We concluded that interpositus cells (and, by extension,RNm) probably controlled some aspect of grasping but were not involved in controlling the reach. Closer examination showed that the rate of discharge typically increased just before the monkey grasped the raisin (or the device handle when the monkey continued the task;the discharge was not specific to the raisin). Examination of video recordings showed that the discharge occurred as the monkey opened his hand to grasp an object.[1,6]
To understand the motor control system, it is necessary to understand the connections between different parts of the brain. We use the neuroanatomical tracer,WGA-HRP, which shows input and output connections of the region of the brain where it is injected. Surprisingly, tracing the connections of RNm to the spinal cord revealed terminations in motor neuronal pools only at lower cervical levels spinal levels C7 and C8 (Fig.3A).
At these spinal levels, motor neurons activate muscles of the hand. The heaviest label from RNm terminated on the motor neurons innervating the extensor digitorum communis (Fig.3B), a muscle that extends the fingers (or toes for a cat). The anatomical tracing nicely agreed with the recording results. The findings suggest that the nucleus interpositus and RNm are specialized for adding hand movements onto limb movements. Surprisingly, a large part of the brain is concerned with only a small part of the reachto-grasp movement.
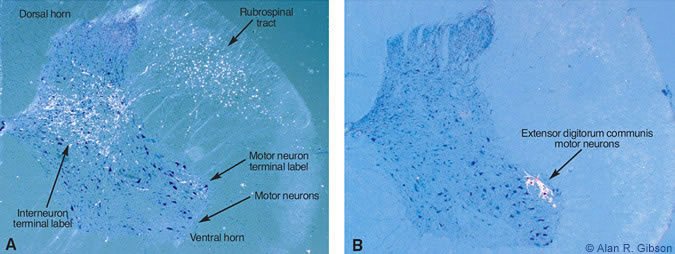
What Part of the Brain Controls Reaching?
If the interpositus nucleus and the RN mainly contribute to the grasp during the reach-to-grasp movement, some other part of the brain must control the limb muscles needed for reaching. The next goal then was to identify regions of the brainstem that might be involved in the control of reach. We first tried to identify brainstem regions that terminate in upper rather than lower cervical spinal segments because the upper segments contain neurons that activate shoulder muscles. This time WGA-HRP was injected into the upper cervical cord, and small neurons in the rostral part of the RN were labeled (Fig. 4).[5] Before this finding,it was believed that the small celled part of the RN projected to the inferior olive and not to the spinal cord.
Cells in RNp Activate Muscles of the Upper Limb
Although the small cells in the RN project to the upper cervical spinal cord, do they actually activate neurons of the upper limb? To answer this question, we electrically activated the fibers connecting RNp to the spinal cord and recorded electrical activity in the muscles. By averaging the muscle recordings using the stimulation pulses as a trigger, activation related to the stimulus pulses can be seen. When stimulated, the fibers from RNp selectively activated muscles of the upper limb and shoulder,[3] thus indicating that the RNp does play a role in control of the upper limb.
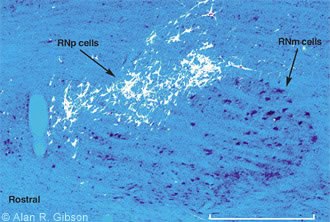
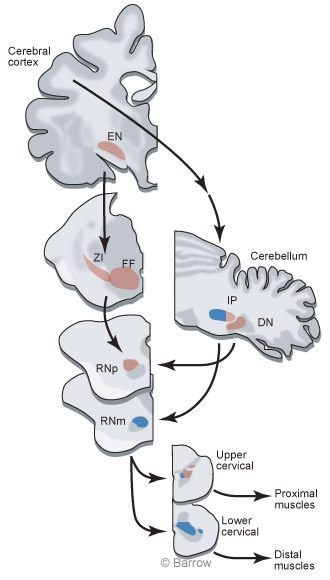
RNp Receives Convergent Input from the Cerebellum and Basal Ganglia
We next injected WGA-HRP into the RNp to determine what parts of the brain supplied input to this region of the RN. Many cells were retrogradely labeled in the dentate nucleus of the cerebellum, indicating that the dentate provided input to RNp. However,many cells were also labeled in more rostral regions of the brain,including the zona incerta and fields of Forel.These inputs were intriguing because we suspected (and confirmed) that output from the basal ganglia terminates in the zona incerta and fields of Forel. The RNp is a region where basal gangliar and cerebellar outputs mix (Fig.5).
We were interested in the convergence of basal gangliar and cerebellar input onto RNp cells because diseases affecting the basal ganglia produce abnormalities in movement. In the most common basal gangliar disease,Parkinson’s disease,cells of the substantia nigra die. Patients with this disease have difficulty in producing movement. Yet, treating a Parkinson’s patient with L-dopa or electrical stimulation in the brain temporarily restores movement. However,neither L-dopa nor deep brain stimulation restores the dead cells in the substantia nigra. Rather, L-dopa and deep brain stimulation alter the output of the basal ganglia.Therefore,it is likely that abnormal output from the basal ganglia interferes with other movement control circuits that are able to produce normal movements. The RN, especially RNp, may be a site where abnormal basal gangliar output can interfere with movements controlled by cerebellar circuits.
Can Basal Gangliar Damage Change Cerebellar Output?
The National Institutes of Health is funding our study of the potential interaction of basal gangliar output with cerebellar circuits. We approached the problem by first producing a chemical lesion in the substantia nigra on one side of the cat brain by injecting 6-OHDA, which selectively kills cells using dopamine as a neurotransmitter. By itself, this damage to the substantia nigra produces no observable change in behavior (Fig.6A).
However, when an excitatory substance (bicuculline, a GABAA antagonist) is injected in or near the RN, the cat’s behavior changes dramatically (Fig. 6B) for a brief time. The motor effects resemble those associated with Parkinson’s disease. As in humans,the cats have reduced spontaneous movement. They move slowly, assume a hunched posture, and sometimes freeze when approaching a corner of the room (Fig.7).
The results suggest that the motor effects of basal gangliar disease may be related to altered excitability of brainstem circuits involved in the cerebellar control of movement — a new view of how basal gangliar diseases influence movement. Our findings are likely to lead to new approaches for treating the motor deficits produced by basal gangliar diseases. After visiting our laboratory, Dr. John Stein at Oxford University and Dr. Tipu Aziz at the Radcliffe Infirmary decided to use our findings to guide them in placing stimulating electrodes in humans to treat severe proximal limb tremor. Their attempt was successful: Stimulation in the areas projecting to the RNp effectively reduced or eliminated proximal tremor.[4] Consequently, our basic research has helped to improve clinical care. Such studies demonstrate how knowledge gained by basic research can lead, often in unanticipated ways, to improvements in medical care.
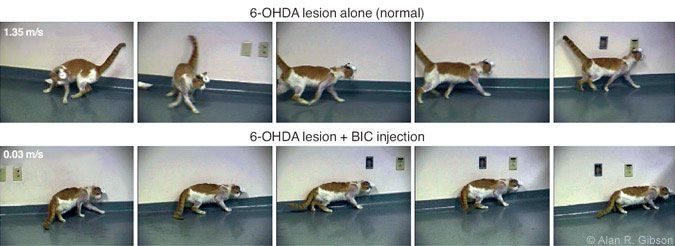

References
- Gibson AR, Horn KM, Stein JF, et al: Activity of interpositus neurons during a visually guided reach. Can J Physiol Pharmacol 74:499-512, 1996
- Gibson AR, Houk JC, Kohlerman NJ: Magnocellular red nucleus activity during different types of limb movement in the macaque monkey. J Physiol 358:527-549, 1985
- Horn KM, Pong M, Batni SR, et al: Functional specialization within the cat red nucleus. J Neurophysiol 87:469-477, 2002
- Nandi D, Chir M, Liu X, et al: Electrophysiological confirmation of the zona incerta as a target for surgical treatment of disabling involuntary arm movements in multiple sclerosis: use of local field potentials. J Clin Neurosci 9:64-68, 2002
- Pong M, Horn KM, Gibson AR: Spinal projections of the cat parvicellular red nucleus. J Neurophysiol 87:453-468, 2002
- Van Kan PL, Horn KM, Gibson AR: The importance of hand use to discharge of interpositus neurones of the monkey. J Physiol 480 ( Pt 1):171-190, 1994