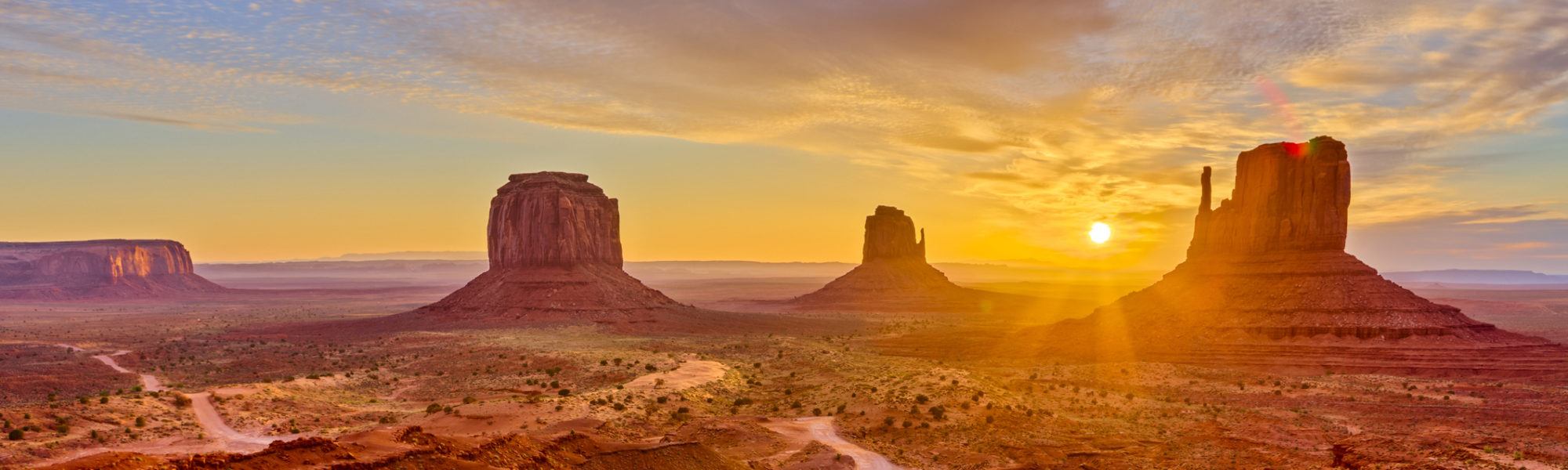
Effect of Melatonin on Cerebral Vasospasm in a Rat Model of Subarachnoid Hemorrhage
Servet Inci, MD*
Joseph M. Zabramski, MD
Robert F. Spetzler, MD
Stephen W. Coons, MD†
*Department of Neurosurgery, School of Medicine, University of Hacettepe, Ankara, Turkey
Divisions of Neurological Surgery and †Neuropathology, Barrow Neurological Institute, St. Joseph’s Hospital and Medical Center, Phoenix, Arizona
Abstract
This study examined the protective effect of melatonin, a powerful oxygen free-radical scavenger, on chronic cerebral vasospasm. Twenty-eight rats were randomly assigned to four groups, seven rats in each group: control, subarachnoid hemorrhage (SAH), SAH plus a placebo, and SAH plus melatonin. SAH was induced by injecting fresh, nonheparinized arterial blood into the cisterna magna. Melatonin and the placebo were administered immediately after SAH and repeated at 12-hour intervals. Animals were sacrificed 96 hours after SAH, and the basilar artery was harvested for morphometric study. Using computerized image analysis, the mean cross-sectional luminal area of the basilar artery in the control group was 58,195 µm2. SAH reduced this parameter by 26% (43,109 µm2) in the untreated and by 30% (40,635 µm2 in the placebo-treated rats compared to the controls. The reduction was similar in the melatonin-treated animals (25%, 43,635 µm2. Despite strong antioxidant properties and the ability to cross the blood-brain barrier, systemic treatment with melatonin did not prevent or significantly attenuate the severity of arterial narrowing in this rat model of chronic cerebral vasospasm. Other spasmogenic agents or a dose-dependent response may have contributed to this unexpected finding.
Key Words: lipid peroxidation, melatonin, oxygen free radicals, rat model, vasospasm
Delayed cerebral vasospasm is a major cause of morbidity and mortality in patients with subarachnoid hemorrhage (SAH).15 Evidence from laboratory and clinical studies suggests that the formation of oxygen free-radicals and the peroxidation of lipids in the cell membrane play an important role in the pathogenesis of cerebral vasospasm.29,30,35,37 After SAH, the auto-oxidation of oxyhemoglobin (OxyHb) to methemoglobin generates superoxide anion radicals and, secondarily, hydrogen peroxide and hydroxyl radicals. These oxygen free radicals initiate and propagate lipid peroxidation according to the Haber-Weis and Fenton reactions.37
The production of oxygen free-radicals is prevalent in cellular metabolism. Under normal conditions, free radicals are rapidly neutralized by a variety of endogenous scavengers, including superoxide dismutase, glutathione peroxidase, and catalase.29 In pathological conditions such as trauma and ischemia, however, this antioxidative defense mechanism is overwhelmed.12
In both in vivo and in vitro studies, the endogenous neuropeptide melatonin (N-acetyl-5-methoxytryptamine) has exhibited protective effects against oxidative tissue damage.6,10,20,33 Melatonin is the most powerful endogenous antioxidant yet isolated.26 It is an effective scavenger of hydroxyl-, peroxyl-, and superoxide radicals23 and exerts its protective effects during free radical-induced cerebral ischemia.5,11 Melatonin also is well tolerated over a wide range of doses and readily crosses the blood-brain barrier.16 Theoretically, free-radical scavengers should be an effective treatment for the prevention of vasospasm. This study was designed to investigate whether melatonin would prevent delayed cerebral vasospasm in a rat model of SAH.
Materials and Methods
The experimental protocol was evaluated and approved by the Animal Research and Ethics Committees of the Barrow Neurological Institute and St. Joseph’s Hospital and Medical Center. Rats were housed in cages (170 x 350 x 175 mm) with one animal per cage. The animal room was windowless with automatic temperature regulation (23 ± 1 C). Access to food and water was free under a 12-hr light/dark cycle (light on at 07:00 AM and off at 07:00 PM).
Experimental Protocol
In this study, 28 male Wistar rats (250-300 g) were randomly assigned to one of four experimental groups (Table 1). All procedures were performed between 10:00 AM and 03:00 PM. Before undergoing procedures, the rats were anesthetized with an intramuscular cocktail of ketamine HCl (4 mg/kg), xylazine (6 mg/kg), and acepromazine maleate (0.8 mg/kg). Anesthesia was maintained as needed by repeated injections of the cocktail at half the above dosage. The left femoral artery was cannulated for monitoring physiological parameters, including heart rate and blood pressure, and for obtaining blood for the subarachnoid injections. The rats were allowed to breathe spontaneously and were given supplemental oxygen (1 L/min). Control animals were not anesthetized and did not undergo surgical exposure.
Subarachnoid Hemorrhage. The rats in experimental groups II, III, and IV had fresh arterial blood injected into their cisterna region to simulate SAH. After anesthesia was induced, the rats were placed prone. Using sterile technique, a small midline incision made at the craniocervical junction was continued caudally until the atlanto-occipital membrane was exposed. Fresh, nonheparinized arterial blood (0.8 ml/kg) was slowly injected into the cisterna magna after an equal amount of cerebrospinal fluid was removed. The rats were kept with their heads down at 20° for 20 minutes to facilitate the blood settling in the basal cisterns. When fully recovered, the rats were returned to their cages and allowed free access to food and water. During the next 4 days, they were observed closely for any deterioration in neurological function.
Treatment Groups. Seven animals were assigned to each treatment group. SAH was not induced in animals in Group 1 (controls). Animals in Group 2 received no additional treatment after blood was injected into the cisterna magna. Animals in Group 3 received a placebo (1% ethanol in saline, 1 ml/kg) intraperitoneally (IP), while rats in Group 4 were given melatonin (10 mg/kg IP). The melatonin (Sigma Chemical Co., St. Louis, MO) was dissolved in absolute ethanol and diluted with saline to a final concentration of 10 mg/ml in a 1% ethanol solution. Treatment with placebo and melatonin was initiated immediately after the blood was injected into the cisterna magna and repeated at 12-hour intervals for 96 hours.
Perfusion-Fixation. The rats in all four experimental groups were sacrificed at 96 hours. After anesthesia was induced, the thorax was opened via a midline sternotomy, and a cannula was placed in the aorta through the left ventricle. The right atrial appendage was opened and the descending aorta was clipped. The cerebrovascular system was flushed under constant pressure with 0.03 M phosphate buffer (50 ml) followed by a fixative solution (100 ml) consisting of 4% paraformaldehyde and 1% glutaraldehyde. After perfusion and fixation, the brains were carefully removed under the operating microscope and placed in the fixative solution for a minimum of 2 days.
Morphometric Analysis
Under the operating microscope, the basilar artery was dissected free of the brain stem. Thin sections of the basilar arteries were cut on a microtome (RMC-MT2C Ultramicrotome, Tucson, AZ) and stained with paragon for morphometric analysis. From each animal, three random arterial cross-sections were measured using computerized image analysis (Kontron Image Analysis System, Munich, Germany) performed by an investigator unaware of the treatment groups. The circumference of the vessel lumen for each of the three sections was averaged for each animal and then used to calculate the maximal cross-sectional area.
Statistical Analysis
The data from the four groups were compared by analysis of variance (ANOVA). Physiological parameters were compared using the paired Student’s t-test.
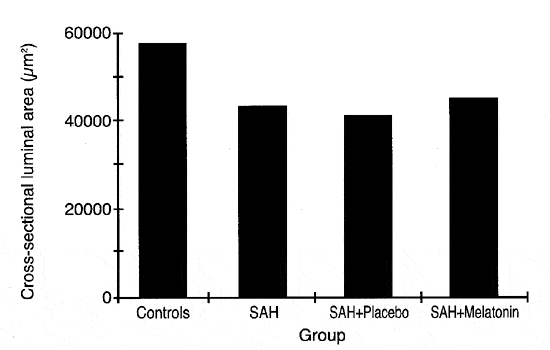
Results
Results
All rats tolerated the procedures well except for one in the placebo group that developed temporary hemiparesis. None of the rats died.
The subarachnoid injection of blood was associated with a nonsignificant increase in heart rate in all animals, from 198 ± 46 to 233 ± 38 beats/min, before and after injection, respectively. Systolic blood pressure was significantly elevated after SAH, measuring 100 ± 4 and 165 ± 4 mm Hg before and after SAH, respectively. Arterial pressure returned almost to normal levels (109 ± 5 mm Hg) 30 minutes after SAH.
The mean cross-sectional luminal area of the basilar artery in the control group was 58,195 ± 20,447 µm2 (Fig. 1). In all of the SAH groups, the mean area of the basilar artery was reduced relative to the control group. In Group II (SAH only), the area of the basilar arteries was reduced by 26% (43,109 ± 19,883 µm2). The percent reduction in cross-sectional luminal area was similar in the placebo-treated animals (Group III, 30%, 40,635 ± 10,039 µm2) and in the melatonin treatment group (Group IV, 25%, 43,635 ± 7,343 µm2).
Discussion
Cerebral vasospasm is a serious complication that adversely affects the outcome of patients with aneurysmal SAH. Since its description by Ecker and Reimenschneider in 1951,7 vasospasm has been studied extensively, yet even its pathogenesis remains controversial. The development of cerebral vasospasm is likely multifactorial. Increasing evidence, however, suggests that this phenomenon, at least partially, reflects the production of oxygen free-radicals and the resulting lipid peroxidation
Sano et al.31 and Sasaki et al.32 originally hypothesized that OxyHb released by the lysis of erythrocytes after SAH might mediate cerebral vasospasm through the generation of oxygen free-radicals and the subsequent peroxidation of membrane lipids. Later studies supported the role of free radicals and lipid peroxidation in the pathogenesis of vasospasm.29,30,34,35,37 Free-radical scavengers have reduced vasospasm in experimental models of SAH and have ameliorated the effects of vasospasm in clinical trials.2,3,9,13,38 Saito et al.28 recently published their results on the effect of ebselen, an antioxidant agent, on 286 patients with aneurysmal SAH enrolled in a multicenter, double-blind, placebo-control trial. Treatment with ebselen reduced brain damage in patients with delayed neurological deficits after SAH.
Melatonin (N-acetyl-5-methoxytryptamine) is the major product of the pineal gland. Its main physiological function is to convey the influence of the light-dark cycle on the body’s physiology and the neuroendocrine system.4 Its full range of physiological functions is unknown. In several recent reports, melatonin acted as a powerful scavenger of hydroxyl, peroxyl, and superoxide radicals.22,23,25 Almost all oxygen free-radicals are scavenged by melatonin. During the process of scavenging free radicals, melatonin is also oxidized to 5-methoxy-N-acetyl-N-formyl-kyn uramine (5-MAFK). The metabolite 5-MAFK likewise exhibits strong antioxidative actions in the Fenton reaction, probably due to its iron-chelating property.22 As an oxygen free-radical scavenger, melatonin is more effective than mannitol, glutathione peroxidase, and vitamin E.1 Furthermore, melatonin stimulates other endogenous antioxidative enzymes, superoxide dismutase, and glutathione peroxidase.24 Because of its high solubility in both lipid and aqueous media, melatonin readily crosses all morphophysiological barriers and enters all subcellular compartments.27
Melatonin may affect the contraction of vascular smooth muscles directly. Takanashi et al.36 and Steele et al.35 demonstrated that the disruption of intracellular Ca++ regulation and overloading are important in the process that causes vasospasm after exposure to oxyhemoglobin. Intracellular calcium binds to calmodulin, and a Ca++-calmodulin complex is formed. This complex then stimulates myosin light-chain kinase, triggering the subsequent phosphorylation of myosin, the generation of cross-linkages between myosin and actin, and the development of tension.40 Melatonin is a calmodulin antagonist that appears to block the formation of the Ca++-calmodulin complex.14 Another calmodulin antagonist, trifluoperazine, provides some protection against vasospasm in dogs.21 These data suggest that melatonin could be effective in the prevention of chronic cerebral vasospasm.
In the present study, however, melatonin had no significant effect on the severity of arterial narrowing in a rat model of SAH. Other mechanisms besides free-radical attack may be involved in vasospasm. About 20 spasmogenic agents identified in the blood, including catecholamines, polypeptides, prostaglandins, and thromboxane A2, cause vasoconstriction in cerebral arteries in vitro.41 Neurogenic immunological and inflammatory factors have also been implicated in the development of vasospasm.17-19
It is also possible that melatonin failed to prevent vasospasm in this model because the dose was inappropriate. In studies of cerebral ischemia, effective doses of melatonin have been in the range of 10 mg/kg/day. We sought to maximize the effect of treatment with melatonin and therefore used a slightly higher dose (20 mg/kg/day). Recently, however, dose-dependent vasoconstrictive effects have been associated with the use of high doses of melatonin in rat cerebral arteries.8,39 This effect is similar to that reported for nitric oxide. At physiological levels nitric oxide mediates vasodilatation but is toxic at high concentrations and can cause injury and arterial vasoconstriction. Consequently, we are investigating the dose-dependent effects of melatonin on cerebrovascular tone.
Conclusions
Theoretically, melatonin is a highly effective free-radical scavenger that should prevent or ameliorate vasospasm. In this study melatonin had no significant effect on the severity of delayed cerebral vasospasm. Further studies, however, are needed to evaluate the dose-dependent effect of this endogenous neuropeptide on cerebrovascular tone.”
References
- Antolín I, Rodríguez C, Sáinz RM, et al: Neurohormone melatonin prevents cell damage: Effect on gene expression for antioxidant enzymes. FASEB J 10:882-890, 1996
- Arthur AS, Fergus AH, Lanzino G, et al: Systemic administration of the iron chelator deferiprone attenuates subarachnoid hemorrhage-induced cerebral vasospasm in the rabbit. Neurosurgery 41:1385-1392, 1997
- Asano T, Takakura K, Sano K, et al: Effects of a hydroxyl radical scavenger on delayed ischemic neurological deficits following aneurysmal subarachnoid hemorrhage: Results of a multicenter, placebo-controlled double-blind trial. J Neurosurg 84:792-803, 1996
- Brzezinski A: Melatonin in humans. N Engl J Med 336:186-195, 1997
- Cho S, Joh TH, Baik HH, et al: Melatonin administration protects CA1 hippocampal neurons after transient forebrain ischemia in rats. Brain Res 755:335-338, 1997
- De La Lastra CA, Cabeza J, Motilva V, et al: Melatonin protects against gastric ischemia-reperfusion injury in rats. J Pineal Res 23:47-52, 1997
- Ecker A, Riemenschneider PA: Arteriographic demonstration of spasm of the intracranial arteries. With special reference to saccular arterial aneurisms. J Neurosurg 8:660-667, 1951
- Geary GG, Krause DN, Duckles SP: Melatonin directly constricts rat cerebral arteries through modulation of potassium channels. Am J Physiol 273:H1530-H1536, 1997
- Germanó A, Imperatore C, D’Avela D, et al: Antivasospastic and brain-protective effects of a hydroxyl radical scavenger (AVS) after experimental subarachnoid hemorrhage. J Neurosurg 88:1075-1081, 1998
- Giusti P, Gusella M, Lipartiti M, et al: Melatonin protects primary cultures of cerebellar granule neurons from kainate but not from N-methyl-D-aspartate excitotoxicity. Exp Neurol 131:39-46, 1995
- Guerrero JM, Reiter RJ, Ortiz GG, et al: Melatonin prevents increases in neural nitric oxide and cyclic GMP production after transient brain ischemia and reperfusion in the Mongolian gerbil (Meriones unguiculatus). J Pineal Res 23:24-31, 1997
- Hall ED: Inhibition of lipid peroxidation in central nervous system trauma and ischemia. J Neurol Sci 134:79-83, 1995
- Horky LL, Pluta RM, Boock RJ, et al: Role of ferrous iron chelator 2,2′-dipyridyl in preventing delayed vasospasm in a primate model of subarachnoid hemorrhage. J Neurosurg 88:298-303, 1998
- Huerto-Delgadillo L, Antón-Tay F, Benítez-King G: Effects of melatonin on microtubule assembly depend on hormone concentration: Role of melatonin as a calmodulin antagonist. J Pineal Res 17:55-62, 1994
- Kassell NF, Torner JC, Haley EC, Jr., et al: The International Cooperative Study on the Timing of Aneurysm Surgery. Part 1: Overall management results. J Neurosurg 73:18-36, 1990
- Lipartiti M, Franceschini D, Zanoni R, et al: Neuroprotective effects of melatonin. Adv Exp Med Biol 398:315-321, 1996
- Macdonald RL: Cerebral vasospasm. Neurosurgery Quarterly 5:73-97, 1995
- Macdonald RL, Weir BKA: A review of hemoglobin and the pathogenesis of cerebral vasospasm. Stroke 22:971-982, 1991
- Macdonald RL, Weir BKA, Grace MGA, et al: Mechanism of cerebral vasospasm following subarachnoid hemorrhage in monkeys. Can J Neurol Sci 19:419-427, 1992
- Pablos MI, Reiter RJ, Chuang J-I, et al: Acutely administered melatonin reduces oxidative damage in lung and brain induced by hyperbaric oxygen. J Appl Physiol 83:354-358, 1997
- Peterson JW, Candia G, Spanos AJ, et al: The calmodulin antagonist trifluoperazine provides mild prophylactic protection against cerebral vasospasm after subarachnoid hemorrhage, but no therapeutic value. Neurosurgery 25:917-922, 1989
- Poeggeler B, Reiter RJ, Tan D-X, et al: Melatonin, hydroxyl radical-mediated oxidative damage, and aging: A hypothesis. J Pineal Res 14:151-168, 1993
- Reiter RJ: Antioxidant actions of melatonin. Adv Pharmacol 38:103-117, 1997
- Reiter RJ, Ortiz GG, Monti MG, et al: Cellular and molecular actions of melatonin as an antioxidant, in Maestroni GJM, Conti A, Reiter RJ (eds): Therapeutic Potential of Melatonin. Basel, Karger, Front Horm Res:81-88, 1997
- Reiter RJ, Pablos MI, Agapito TT, et al: Melatonin in the context of the free radical theory of aging. Ann N Y Acad Sci 786:362-378, 1996
- Reiter RJ, Poeggeler B, Tan D-X, et al: Antioxidant capacity of melatonin: A novel action not requiring a receptor. Neuroendocrinol Lett 15:103-116, 1993
- Reiter RJ, Tan D-X, Poeggeler B, et al: Melatonin as a free radical scavenger: Implications for aging and age-related diseases. Ann N Y Acad Sci 719:1-12, 1994
- Saito I, Asano T, Sano K, et al: Neuroprotective effect of an antioxidant, ebselen, in patients with delayed neurological deficits after aneurysmal subarachnoid hemorrhage. Neurosurgery 42:269-278, 1998
- Sakaki S, Kuwabara H, Ohta S: Biological defence mechanism in the pathogenesis of prolonged cerebral vasospasm in the patients with ruptured intracranial aneurysms. Stroke 17:196-202, 1986
- Sakaki S, Ohta S, Nakamura H, et al: Free radical reaction and biological defense mechanism in the pathogenesis of prolonged vasospasm in experimental subarachnoid hemorrhage. J Cereb Blood Flow Metab 8:1-8, 1988
- Sano K, Asano T, Tanishima T, et al: Lipid peroxidation as a cause of cerebral vasospasm. Neurol Res 2:253-272, 1980
- Sasaki T, Wakai S, Asano T, et al: The effect of a lipid hydroperoxide of arachidonic acid on the canine basilar artery. An experimental study on cerebral vasospasm. J Neurosurg 54:357-365, 1981
- Sewerynek E, Reiter RJ, Melchiorri D, et al: Oxidative damage in the liver induced by ischemia-reperfusion: Protection by melatonin. Hepatogastroenterology 43:898-905, 1996
- Shishido T, Suzuki R, Qian L, et al: The role of superoxide anions in the pathogenesis of cerebral vasospasm. Stroke 25:864-868, 1994
- Steele JA, Stockbridge N, Maljkovic G, et al: Free radicals mediate actions of oxyhemoglobin on cerebrovascular smooth muscle cells. Circ Res 68:416-423, 1991
- Takanashi Y, Weir BKA, Vollrath B, et al: Time course of changes in concentration of intracellular free calcium in cultured cerebrovascular smooth muscle cells exposed to oxyhemoglobin. Neurosurgery 30:346-350, 1992
- Takenaka K, Kassell NF, Foley PL, et al: Oxyhemoglobin-induced cytotoxicity and arachidonic acid release in cultured bovine endothelial cells. Stroke 24:839-846, 1993
- Toyoda T, Kwan A-L, Bavbek M, et al: Enhanced endogenous antioxidant activity and inhibition of cerebral vasospasm in rabbits by pretreatment with a nontoxic endotoxin analog, monophosphoryl lipid A. J Neurosurg 88:1082-1087, 1998
- Viswanathan M, Scalbert E, Delagrange P, et al: Melatonin receptors mediate contraction of a rat cerebral artery. Neuroreport 8:3847-3849, 1997
- Vollrath BAM, Weir BKA, Macdonald RL, et al: Intracellular mechanisms involved in the responses of cerebrovascular smooth-muscle cells to hemoglobin. J Neurosurg 80:261-268, 1994
- White RP: Responses of isolated cerebral arteries to vasoactive agents. Neurosurg Clin North Am 1:401-415, 1990